Summary
The aims of most seismological applications are to unravel information about the Earth’s interior, at depths that cannot be probed or observed directly. In most applications of signal detection, processing, and subsequent seismic imaging, seismic noise is considered a nuisance that obscures the signal from recorded sources such as earthquakes or explosions. However, more recent advances in observational seismology have proven that noise is in fact an extremely valuable resource that can be used to our advantage. Not only it contains information about the medium of propagation of waves from a wide range of continuous and simultaneous sources in the ground, but it can be manipulated such that we can create desired configurations of virtual sources and receivers to satisfy the much needed input for various processing algorithms [1,2] . This is in stark contrast to seismic imaging techniques that depend on active seismic sources such as local earthquakes, vibroseis, or dynamite, to create images of the Earth’s subsurface. The location of these sources is not always controllable and is heterogeneously distributed in both time and space, with a limited azimuthal coverage, thus heavily biasing the output images [3] The basic concept for turning noise into signal is cross-correlation and stacking of extensive waveform data recorded at pairs of ground motion detectors [4]. What is most remarkable by this apparently simple approach is that by stacking days or months of cross-correlated noise, one obtains a waveform that equates a high quality signal emitted as if a source was placed at one of the receivers [5]. In other words, one would transform a receiver into a virtual source. This has fundamental implications into the abilities of seismic imaging and has gained increasing popularity in observational seismology in the past decade [6,7,8]. The only requirement is having a wide range of receivers that record noise simultaneously for a long enough time to extract high quality signals that can be subsequently manipulated in the same way as classic seismic data, and, of course, the necessary technical tools to process them.
The National Institute for Earth Physics (NIEP) has been maintaining a permanent network of broadband seismic stations for decades [9], as well as temporary networks that were operational for months to years, spanning the Carpathian-Pannonian region (Figure 1) [10,11]. The high quality and the longevity of the recordings archived at NIEP makes the dataset an excellent candidate for applying the most recent techniques for ambient noise analysis and for further processing to obtain information on the Earth’s interior. Specifically, we wish to analyse the structure of the crust (0-40 km) of the entire Carpathian-Pannonian region using cross-correlated waveform data, by applying the most novel techniques of anisotropic seismic velocity tomography, imaging the 3D absorption, near surface geophysics and elastic scattering of seismic waves inside the Pannonian and Transylvanian Basins, the Carpathian Orogen, and its foreland regions. We will thus benefit from
the existence of large sets of observation data which are available at present in this area.
The Carpathian-Pannonian region is an ideal natural laboratory to study processes related to extensional basin evolution, oceanic subduction, post-collisional volcanism, a well as active crustal deformation associated with the push of the Adria plate [12] or the pull of the actively detaching Vrancea slab [13]. The geological history includes the subduction of the Tethys Ocean whose ophiolitic remnants are buried beneath thick sedimentary covers, the collision of multiple microplates with the East European Craton in the past 20 Ma, culminating with the formation of the arcuate Carpathian orogenic system [14,15] beneath which active slab break-off generates high magnitude earthquakes in the Vrancea Seismic Zone (15,VSZ). The VSZ is known to produce highmagnitude earthquakes every 30-40 years [17] and releases the highest strain in continental Europe [18] but the way earthquakes are felt are highly anomalous: instruments tend to record higher peak ground accelerations further away from the epicenter [19] and seismic waves appear to be highly attenuated towards the NW in the Transylvanian area [20], while most of the Carpathian foreland and surrounding regions are more susceptible to destructive shaking [21] due to the complex seismic response of the geological structure [22]. These anomalous features have yet to be explained and are strongly related to how waves propagate through the heterogeneities and anisotropic structures inside the Earth. Improving our understanding of the Earth’s architecture in this area of the world will bring us closer to determining the reasons for the complex anomalous behaviour of the seismic wavefield of real earthquakes generated by the Vrancea slab [23]. Studying the properties of the crust around this earthquake-prone region is of utmost importance to improve the assessment of earthquake hazard and seismic risk, and also to potentially improve the way earthquake engineering agents operate in developing socio-economic regions of Eastern Europe that are especially vulnerable to natural hazards.
Objectives
Mitigate the negative effects of uneven seismic source distribution, vital for high-resolution seismic imaging, by creating “virtual” sources at the location of every available seismic station in Eastern Europe with the help of recorded noise. The obtained waveforms will be used to create an exhaustive dataset of cross-correlograms which will serve as the starting base for a range of seismic imaging processing techniques that will image the crustal and upper mantle structures of Eastern Europe at the highest possible resolution and with the least amount of numerical artifacts introduced previously by uneven source distribution and insufficient azimuthal ray coverage.
Apply state-of-the-art ambient noise seismic imaging techniques to illuminate different physical properties of the crust in Eastern Europe at the highest possible resolution and with the least amount of numerical artifacts introduced previously by uneven source distribution and insufficient azimuthal ray coverage. These include scattering and absorption studies [20], anisotropic seismic tomography [32], and near-surface geophysics techniques [22,23]. Most importantly, the inclusion of an anisotropy component into the inversion of the data is of utmost importance to minimise the artifact anomalies associated with isotropic-only velocity models. The continuous and simultaneous source distribution that characterises noise allows for an excellent azimuthal coverage, previously unrecognised, and for the most accurate and artifact-free retrieval of anisotropic properties.
Gain fundamental insights into the structure, deformation, and geological processes that shaped or are actively reworking the crust of the Pannonian and Transivanian sedimentary basins, and the Carpathian Orogen. Our techniques allow us to illuminate buried geological structures with minimal numerical bias at a regional scale, including the depth extent of major geological faults and intracrustal discontinuities, volcanoes or magmatic intrusions, ocean ophiolites from the subduction of Tethys and its eastern oceanic branches [14,15], or sedimentary layers.
Improve our understanding of the anomalies associated with the Vrancea Seismic Zone, the highest strain-releasing seismogenic body in Europe [31]. These include the uneven distribution of seismic intensities [21] and the anomalous increase of peak ground acceleration with increasing distance from the epicentral area, all of which are undoubtedly related to the crustal heterogeneous and anisotropic structures seismic waves propagate through.
Funding information
Funding | UNITATEA EXECUTIVA PENTRU FINANTAREA INVATAMANTULUI SUPERIOR, A CERCETARII, DEZVOLTARII SI INOVARII (UEFISCDI) |
---|---|
PNIII | National research, development and innovation plan 2015-2020 |
Program name from PNIII | P4 – Fundamental and frontier research |
Project type | Exploratory research projects (PCE)PN-III-ID-PCE-2020-3 |
Project title | Eastern European Ambient Seismic Noise (EENSANE) |
Total contract value | 1 197 532.00 RON |
Contract length | 36 months |
Project begin date | 12/02/2021 |
Project end date | 31/01/2024 |
Principal investigator | LAURA PETRESCU (laura.petrescu@infp.ro) |
Informatii financiare
FINANŢARE | UNITATEA EXECUTIVA PENTRU FINANTAREA INVATAMANTULUI SUPERIOR, A CERCETARII, DEZVOLTARII SI INOVARII (UEFISCDI) |
---|---|
PNIII | PLANUL NAŢIONAL DE CERCETARE, DEZVOLTARE ŞI INOVARE, 2015-2020 |
Denumirea Programului din PN III | P4 – CERCETARE FUNDAMENTALĂ ȘI DE FRONTIERĂ |
Tip proiect | PROIECTE DE CERCETARE EXPLORATORIE (PCE)PN-III-ID-PCE-2020-3 |
Titlul proiectului | Zgomot versus semnal: imagistica seismic de frontiera in Estul Europei |
Valoarea totală a Contractului | 1.197.532,00 lei |
Durata contractului | 36 luni |
Data de începere a proiectului | 12/02/2021 |
Data de finalizare a proiectului | 31/01/2024 |
Director Proiect EENSANE | LAURA PETRESCU (laura.petrescu@infp.ro) |
Project timeline
This project is split in four concurrent work packages over approximately 3 years. Below is a Gantt chart of our activities.
Expected results
The key results planned in the project (3D seismic structure, attenuation and anisotropy properties) are essential in the evaluation of local site amplification and regional seismic hazard and risk assessment, and civil engineering applications such as earthquake engineering building design [23]. These are especially important in socio-economic developing countries with high vulnerability to natural hazards such as the high-magnitude earthquakes generated in the Vrancea Seismic Zone [16]. In areas with low seismicity, such as the Pannonian Basin, these results might refine the building code of the countries within this region. On a more fundamental level, our results can improve our understanding of past geological phenomena that shaped the Eastern European region such as the Tethys oceanic subduction, continental collision, buried volcanic features, and sedimentary basin evolution, as well as past and present plate kinematics through the use of anisotropy as proxy [14,15]. These results may provide key constraints for other disciplines such as evolutionary biology research related to landscape changes, earthquake generation forecasting research based on active deformation, or even climate change as affected by topography or by past or potentially active volcanism in the intra-Carpathian region.
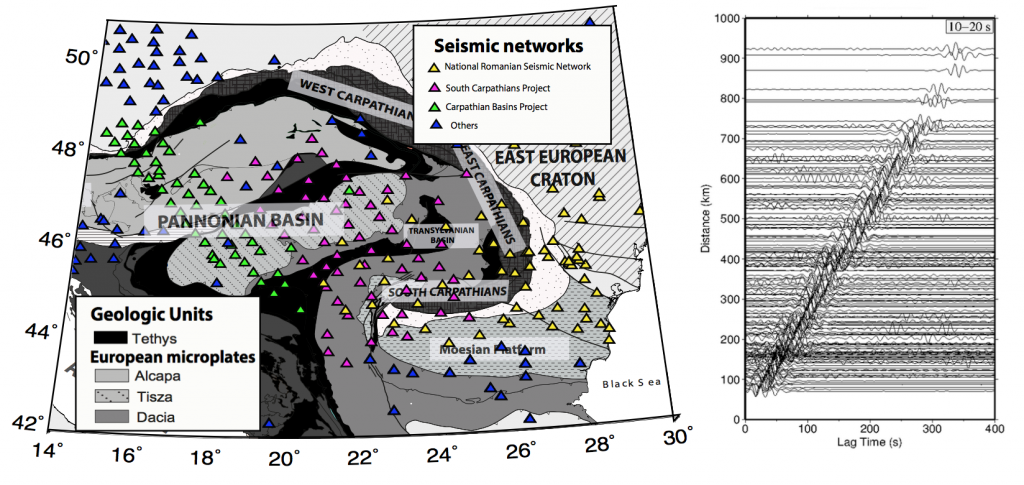
References
1. Curtis, A., Gerstoft, P., Sato, H., Snieder, R. and Wapenaar, K., 2006. Seismic interferometry—Turning noise into signal. The Leading Edge, 25(9), pp.1082-1092.
2. Fichtner, A., Stehly, L., Ermert, L. and Boehm, C., 2016. Generalised interferometry-I. Theory for inter-station correlations. Geophysical Journal International, p.ggw420.
3. Rawlinson, N., Fichtner, A., Sambridge, M. and Young, M.K., 2014. Seismic tomography and the assessment of uncertainty. In Advances in Geophysics (Vol. 55, pp. 1-76). Elsevier.
4. Bensen, G.D., Ritzwoller, M.H., Barmin, M.P., Levshin, A.L., Lin, F., Moschetti, M.P., Shapiro, N.M. and Yang, Y., 2007. Processing seismic ambient noise data to obtain reliable broad-band surface wave dispersion measurements. Geophysical Journal International, 169(3), pp.1239-1260.
5. Curtis, A., Nicolson, H., Halliday, D., Trampert, J. and Baptie, B., 2009. Virtual seismometers in the subsurface of the Earth from seismic interferometry. Nature Geoscience, 2(10), pp.700-704.
6. Yang, Y., Ritzwoller, M.H., Levshin, A.L. and Shapiro, N.M., 2007. Ambient noise Rayleigh wave tomography across Europe. Geophysical Journal International, 168(1), pp.259-274.
7. Li, H., Li, S., Song, X.D., Gong, M., Li, X. and Jia, J., 2012. Crustal and uppermost mantle velocity structure beneath northwestern China from seismic ambient noise tomography. Geophysical Journal International, 188(1), pp.131-143.
8. Green, R.G., Priestley, K.F. and White, R.S., 2017. Ambient noise tomography reveals upper crustal structure of Icelandic rifts. Earth and Planetary Science Letters, 466, pp.20-31.
9. Neagoe, C., Manea, L.M., Marmureanu, A. and Ionescu, C., 2019, January. A Review of Seismic Monitoring in Romania: improved earthquake detection network capabilities. In Geophysical Research Abstracts (Vol. 21).
10. Ren, Y., Stuart, G.W., Houseman, G.A., Dando, B., Ionescu, C., Hegedüs, E., Radovanović, S., Shen, Y. and South Carpathian Project Working Group, 2012. Upper mantle structures beneath the Carpathian–Pannonian region: implications for the geodynamics of continental collision. Earth and Planetary Science Letters, 349, pp.139-152.
11. Dando, B.D.E., Stuart, G.W., Houseman, G.A., Hegedüs, E., Brückl, E. and Radovanović, S., 2011. Teleseismic tomography of the mantle in the Carpathian-Pannonian region of central Europe. Geophysical Journal International, 186(1), pp.11-31.
12. Bada, G., Horváth, F., Dövényi, P., Szafián, P., Windhoffer, G. and Cloetingh, S., 2007. Present day stress field and tectonic inversion in the Pannonian basin. Global and Planetary Change, 58(1-4), pp.165-180.
13. Sperner, B., F. Lorenz, K. Bonjer, S. Hettel, B. Muller, and F. Wenzel (2001), Slab break-off–abrupt cut or gradual detachment? New insights from the Vrancea Region (SE Carpathians, Romania), Terra Nova, 13(3), 172–179, doi:10.1046/j.1365-3121.2001.00335.x.
14. Schmid, S. M., D. Bernoulli, B. Fu ̈genschuh, L. Matenco, S. Schefer, R. Schuster, M. Tischler, and K. Ustaszewski (2008), The Alpine-Carpathian-Dinaridic orogenic system: correlation and evolution of tectonic units, Swiss J. Geosci., 101(1), 139–183, doi:10.1007/s00015 008- 1247-3.
15. Ustaszewski, K., S. M. Schmid, B. Fugenschuh, M. Tischler, E. Kissling, and W. Spakman (2008), A map-view restoration of the Alpine-Carpathian-Dinaridic system for the early Miocene, Swiss J. Geosci., 101(SUPPL. 1), 273–294, doi:10.1007/s00015-008-1288-7.
16. Ismail-Zadeh, A., L. Matenco, M. Radulian, S. Cloetingh, and G. Panza (2012), Geodynamics and intermediate-depth seismicity in Vrancea (the south-eastern Carpathians): current state- of-the art, Tectonophysics, 530, 50–79, doi:doi.org/10.1016/j.tecto.2012.01.016
17. Radulian, M., Popa, M., Cărbunar, O. and Rogozea, M., 2008. Seismicity patterns in Vrancea and predictive features. Acta Geodaetica et Geophysica Hungarica, 43(2-3), pp.163 173.
18. Oncescu, M.-C., and K.-P. Bonjer (1997), A note on the depth recurrence and strain release of large Vrancea earthquakes, Tectonophysics, 272(2-4), 291–302, doi:10.1016/S0040- 1951(96)00263-6
19. Mărmureanu, G., Mărmureanu, A., Florinela, E., Manea, D.T.D. and Vlad, M., 2016. Can we still use classic seismic hazard analysis for strong and deep Vrancea earthquakes?. Romanian Journal of Physics, Romania, 61(3-4), pp.728-738.
20. Borleanu, F., De Siena, L., Thomas, C., Popa, M. and Radulian, M., 2017. Seismic scattering and absorption mapping from intermediate-depth earthquakes reveals complex tectonic interactions acting in the Vrancea region and surroundings (Romania). Tectonophysics, 706, pp.129-142.
21. Sokolov, V., Bonjer, K.P., Wenzel, F., Grecu, B. and Radulian, M., 2008. Ground-motion prediction equations for the intermediate depth Vrancea (Romania) earthquakes. Bulletin of Earthquake Engineering, 6(3), pp.367-388.
22. Manea, E.F., Michel, C., Poggi, V., Fäh, D., Radulian, M. and Balan, F.S., 2016. Improving the shear wave velocity structure beneath Bucharest (Romania) using ambient vibrations. Geophysical Journal Internationall, 207(2), pp.848-861.
23. Manea, E.F., Michel, C., Hobiger, M., Fäh, D., Cioflan, C.O. and Radulian, M., 2017. Analysis of the seismic wavefield in the Moesian Platform (Bucharest area) for hazard assessment purposes. Geophysical Journal International, 210(3), pp.1609-1622.
24. Ren, Y., Grecu, B., Stuart, G., Houseman, G., Hegedüs, E. and South Carpathian Project Working Group, 2013. Crustal structure of the Carpathian–Pannonian region from ambient noise tomography. Geophysical Journal International, 195(2), pp.1351-1369.